The Next 10 Years
Section contributors: Edgard G. Rivera Valentín, Joseph G. O'Rourke, Ramses Ramirez, Maitrayee Bose, Kunio M. Sayanagi, and Brett Denevi.
An Introduction to the Decadal Survey
We sometimes take for granted the bureaucratic processes that turn ideas for space missions into actual flight hardware. For NASA, that process is the decadal survey, a report produced every 10 years by the scientific community for each of NASA’s 4 science divisions. The current decadal survey for planetary science was released in 2011 and set priorities for 2013 to 2022. Work on the next iteration, covering 2023 through 2032, formally kicks off this year.
The value of the decadal surveys comes from their open, deliberative process among various planetary science constituencies. Through a process overseen by the National Academy of Sciences, scientists who study specific aspects of the solar system such as the Moon or the outer planets settle on the big science questions for their field and which space missions should answer those questions.
Recommendations made in the decadal reports tend to happen despite the fact that the reports are advisory. Congress, finding the decadal process helpful for evaluating NASA’s funding requests, passed legislation making those recommendations an official part of NASA’s policy-setting process. As a consequence, the decadal survey serves 2 major purposes: to drive resources into new projects and to defend the cancellation of previously endorsed missions.
In the early 2010s, NASA’s Planetary Science Division faced its largest cuts in 30 years. The Planetary Society, working hand in hand with other professional organizations, rallied around the decadal survey to defend existing missions and argue for increased funding for recommended ones. Today, the planetary science budget has rebounded, reaching levels not seen since the days of the Viking missions to Mars in the 1970s. NASA’s Mars 2020 and Europa Clipper spacecraft are moving toward launch. The Dragonfly mission to Titan is in development, and the lower-cost InSight, Lucy, and Psyche missions were selected to explore Mars and asteroids.
As work on the next decadal survey kicks off, we’ve asked scientists representing Mercury, Venus, the Moon, Mars, small bodies, and the outer planets to tell us what we’ve learned in the past decade and to give us 3 big questions that will drive future missions for the next decade and beyond. The planning, debate, and effort for missions to answer those questions is already in progress. Who knows what marvelous scientific discoveries await as a result?

Mercury, the first planet from the Sun, is an enigmatic world with important insights for planetary scientists. It has water ice at its poles, which was discovered by the Arecibo Observatory in Puerto Rico and the Goldstone Solar System Radar. This is despite the fact that Mercury’s surface can reach 430-degrees Celsius (800-degrees Fahrenheit). The planet has a self-generated magnetic field created by a molten core even though it’s only about 3 times the volume of our Moon.
NASA’s MESSENGER spacecraft orbited Mercury from 2011 to 2015 and revealed that the planet’s core comprises nearly 85 percent of the planet’s volume, hinting at a unique formation history. The surface has been heavily modified by volcanoes and tectonics and may still be active today as Mercury contracts. MESSENGER also saw chemically distinct surface features that we still don’t fully understand. These findings have led us to fundamental questions about Mercury that, if answered, would help us better understand important planetary processes:
How did Mercury form?
How did Mercury get such a large core?
From where did Mercury’s polar ice originate, and how is it distributed in the planet’s subsurface?
BepiColombo, a joint mission between Europe and Japan, launched to Mercury in 2018 and will arrive in 2025. It will study the planet for at least 2 years. Studying Mercury’s interior and unraveling its early history will help us understand how it formed and thus provide insights on the formation of our solar system. We can also learn how Mercury generates a magnetic field, which in turn will help us better understand how planetary magnetic fields form and why some planets have them while others don’t. Mercury is also an excellent laboratory to refine our understanding of how stars interact with planetary surfaces via space weathering.
— EDGARD G. RIVERA-VALENTÍN is a staff scientist with Universities Space Research Association at the Lunar and Planetary Institute. Asteroid (389478) 2010 ER87 is named after them.

Venus is perhaps the most Earth-like world in the solar system. However, only 3 space missions have targeted Venus in the past 30 years. They provided tantalizing hints that Venus, though hellish today, maintained temperate surface conditions for billions of years—much longer than Mars! Venus also holds the key to interpreting ongoing observations of Venus- and Earth-sized exoplanets.
Magellan, the last NASA-led mission to Venus, arrived on 10 August 1990. (I was 10 days old.) The spacecraft mapped the surface with radar to see through the thick, visibly opaque atmosphere. Venus is volcanically active today, with an average surface age under 1 billion years.
Missions after Magellan, 2 of which operated in the past decade, focused on atmospheric dynamics. Europe’s Venus Express operated from 2006 to 2014, while Japan’s Akatsuki failed to enter orbit in 2010 but succeeded in 2015 and survives today. This plucky spacecraft discovered a huge, stationary gravity wave and mapped 3D wind speeds.
The Venus community recently developed a consensus list of scientific goals to inform the next decadal survey. Here are 3 of the many high-priority questions:
Did Venus ever host oceans?
Venus Express discovered that ancient, highly deformed terrain called tesserae may have granite-like compositions, which would imply that they formed near abundant liquid water. The next Venus orbiter could confirm this by measuring the spectral signatures of tesserae with an instrument optimized for the task. Follow-up tesserae landers could characterize their mineralogy in detail.
Can the evolution of Venus help us understand the evolution of exoplanets?
Beyond chasing surface water, we need to narrow down the fundamental properties of Venus that control its evolution as a planetary system over time. In particular, any spacecraft that visits the deep atmosphere should measure the abundances and isotopic ratios of noble gases like krypton and xenon for comparison to well-known values found on Earth and Mars. These measurements will help us learn how Venus got its atmosphere and how certain compounds like water were lost to space over time.

What geologic processes have shaped the surface of Venus?
Whether Venus’ crust was resurfaced in a catastrophic event or more gradually has been debated since Magellan. Our existing maps have limited resolution, similar to what we had for Mars in the 1970s. Higher-resolution images would permit basic geologic mapping: identification of different rock types, their distributions on the surface, and how they aged over time. Additionally, better imagery and topography for the entire surface could reveal why Venus lacks plate tectonics.
A mission in NASA’s low-cost Discovery flight line, equipped with a modern radar and an infrared camera customized for Venus, would answer most of these high-priority questions while leaving some (including those not listed above) unanswered. Given its potential long-lasting habitability and implications for terrestrial exoplanets, Venus deserves to have a multimission program started in the next decade.
—JOSEPH G. O’ROURKE is an assistant professor in the School of Earth and Space Exploration at Arizona State University and serves on the steering committee of NASA’s Venus Exploration Analysis Group.

For the Moon, our partner in a billions-year- long dance, the 2010s were a decade of profound revelations. Discoveries from our robotic spacecraft and a closer look at soil and rock samples collected by astronauts during the Apollo missions have led lunar scientists to a new understanding of the Moon.
Starting in 2009, NASA launched 4 missions to the Moon: Lunar Reconnaissance Orbiter and its crater-creating partner, LCROSS; GRAIL, which gave us new maps of the Moon’s gravity field; and the dust-detecting LADEE. China placed the Chang’e-3 and -4 landers and rovers on the surface, while India launched Chandrayaan-2 into lunar orbit.
We confirmed the existence of water ice in the Moon’s permanently shadowed polar regions. We found water locked in Apollo moon rocks in abundances that were surprising given the Moon’s violent and fiery origin. Enormous dikes, visible only in gravity data, may have provided pathways to the surface for the floods of lavas that covered much of the nearside. The Moon’s volcanism, while mostly ancient, may have continued up to less than 100 million years ago, meaning our small Moon retained enough heat to sustain eruptions through essentially the present day, geologically speaking.
With new discoveries comes the ability to ask new questions or find new ways to think about old ones. Fortunately, the lunar community is ready, armed with a wealth of data and an enthusiastic, vibrant group of scientists. Our high-priority questions for the coming decade include:
What does the Moon’s history tell us about the time period for the late heavy bombardment, when the inner solar system was battered with asteroids and comets?
What is the structure and composition of the Moon’s interior?
What is the nature and origin of water and other volatiles at the Moon’s poles, and what is the history of magmatism during more than 4 billion years of lunar evolution?
The South Pole–Aitken Basin Sample Return mission, which appeared in both the 2003-to-2012 and 2013-to-2022 decadal surveys, would bring rocks and regolith from an unexplored region of the Moon back to Earth. By dating the formation of the largest basin on the Moon, we would gain vital information to constrain the period of late heavy bombardment—a period when Earth and the Moon were slammed with comets and asteroids, the end of which may coincide with the emergence of life on Earth.

The Lunar Geophysical Network, which appeared in the current decadal survey, would see several landers widely distributed across the lunar surface acquire simultaneous seismic and heat-flow measurements. This network would tell us about the structure of the Moon’s mantle and core as well as its thermal state and evolution and inform our understanding of all rocky, differentiated worlds that are separated into distinct layers beneath the surface.
Rovers that could travel long distances and operate through the lunar night or in the extreme cold of permanent shadows would allow us to answer a multitude of key planetary science questions. A rover measuring the composition and abundance of the Moon’s water and other volatiles at its poles would provide a window to how and when water was delivered to the Earth- Moon system billions of years in the past. A rover exploring Oceanus Procellarum, the largest dark region you can see on the Moon’s nearside from Earth, would give us access to 4 billion years of lunar volcanism and a huge diversity of landforms, compositions, and eruption styles.
—BRETT DENEVI is a planetary geologist at the Johns Hopkins University Applied Physics Laboratory. She is currently serving as the deputy principal investigator for the Lunar Reconnaissance Orbiter camera. Asteroid 9026 is named Denevi in her honor.

Mars science has come a long way since Percival Lowell looked through his telescope and saw what he thought were irrigation canals on the Red Planet. To this day, the question of whether life exists or ever existed there remains one of the primary drivers of Mars exploration.
In the past decade, NASA’s Curiosity rover discovered organic compounds—the building blocks of life as we know it—on the surface. Curiosity also sniffed out seasonal releases of methane, which could be linked to underground life. The European Space Agency’s Mars Express orbiter found evidence for a possible lake underneath the Martian south pole. This is all consistent with new laboratory experiments and fieldwork suggesting that Mars’ subsurface may be able to host thriving microbial communities.
We know that ancient Mars had rivers, deltas, and lakes on its surface, supporting the idea of a warmer past climate. Analyses of NASA’s MAVEN spacecraft data suggest that the planet likely lost at least 0.5 bar of atmosphere—roughly half of the pressure of Earth’s atmosphere at sea level—over the past 4 billion years. (It could be as high as 1 bar or more.) However, we still don’t know what Mars was like in its early days. Fortunately, new missions and laboratory work over the next decade could help us figure that out. The answers have implications far beyond Mars: if life emerged independently on 2 planets in our solar system, then it is more likely to be relatively common throughout the cosmos.
What was the atmospheric composition of a warmer early Mars?
One of the great mysteries of Mars’ early history is understanding the atmospheric composition that would have supported the once-warmer and once-wetter climate. Because the Sun was much fainter 4 billion years ago, Mars couldn’t have been warmed up by just carbon dioxide and water, the principal greenhouse gases we have here on Earth. Recent models suggest that early Mars could have had a predominantly carbon-dioxide-and-hydrogen- based atmosphere (along with water) instead, which is consistent with our having yet to observe iron bands in Martian rocks that would have likely formed in a more oxygen-rich atmosphere like that on Earth.
We need to run experiments to evaluate whether a predominantly carbon dioxide and hydrogen atmosphere would alter a planet’s surface in a way that matches what we see on Mars. Then, future Mars missions, including human crews, could assess rock layers and compare them with our laboratory experiments.

Was early Mars warm and wet or cold and icy with temporary episodes of warmth triggered by events like short-lived volcanism and meteoritic impacts?
NASA’s Mars 2020 rover will examine an ancient river delta in Jezero crater. There, it can search for frost wedges—places where rocks fracture due to persistent cold temperatures. The presence of frost wedges would support the cold-and-icy theory. Likewise, Mars 2020 will search Jezero’s rock layers for signs of a long-term lake, which would be consistent with a persistently warm and wet climate.
Did life exist—or does life currently exist—on Mars?
A warmer ancient Mars with a thicker atmosphere could have facilitated the emergence of life. If so, fossils may be preserved today. Although the present Martian surface is sterile, life may be safely living in the subsurface. NASA’s Mars 2020 rover and the European Space Agency’s Rosalind Franklin rover are both scheduled to launch this year. They are equipped with Raman spectrometers that can detect organic compounds and decode their structures. These instruments alone won’t confirm the existence of life, but they could point us in the right direction, especially for determining which samples Mars 2020 should store for future return to Earth for more careful analysis.
—RAMSES RAMIREZ is a planetary scientist and astrobiologist from the Earth-Life Science Institute at the Tokyo Institute of Technology and a Space Science Institute affiliate scientist.

Multiple missions to our solar system’s smallest worlds ushered in a new era of smallbody science during the last decade. Europe’s Rosetta spacecraft flew past 2 asteroids before arriving at comet Churyumov-Gerasimenko. China’s Chang’e-2 visited asteroid Toutatis in 2012. NASA’s Stardust mission, having encountered comet Wild 2 in 2004, was repurposed to fly past comet Tempel 1 in 2011.
There were also 3 sample return missions, 2 of which are still in progress. Japan’s Hayabusa spacecraft returned samples from asteroid Itokawa to Earth in 2010. Hayabusa2 is on its way back to Earth with samples from Ryugu, while NASA’s OSIRIS-REx is surveying asteroid Bennu in preparation to collect a sample in August 2020.
We have learned that Itokawa, Ryugu, and Bennu are all “rubble-pile” asteroids— essentially, rocks and boulders loosely held together by gravity. The diamond shapes of Bennu and Ryugu indicate there are common processes altering these worlds, including the YORP effect, which alters an asteroid’s spin rate due to solar heating and thermal emission. Studies suggest this effect could be responsible for separating some top-shaped asteroids into 2 objects.
Beyond Neptune, NASA’s New Horizons spacecraft visited 2 Kuiper Belt objects: Pluto and Arrokoth. The almost-perfectly round lobes of Arrokoth are likely a result of 2 separate spheres of boulders and rocks clumping together and then colliding at slow speeds. This tells us that slow-speed impacts may have been a common occurrence in the early solar system.
Also in the past decade, we discovered additional moons around Jupiter. Many have eccentric, highly inclined orbits—typically opposite from the direction of Jupiter’s rotation—which tells us they were probably captured by Jupiter’s gravity rather than forming near the planet.
Our myriad discoveries in the last decade have reaffirmed our need to study small bodies in detail. Missions and investigations in the next decade will hopefully answer several of our big-picture questions, which include:
Are asteroids and comets primordial bodies, meaning they are building blocks of planets?
If asteroids and comets are the precursor objects that formed the larger planets by accretion, then it’s essential that we keep exploring them for ingredients essential to life, such as water, amino acids, sugars, and ketones. We also need to understand the processes that shaped these worlds into how they appear today. The samples returned by OSIRIS-REx and Hayabusa2 could help immensely. Additionally, their detailed analyses using state-of-the-art laboratory techniques with high accuracy and precision will enable us to answer the big-picture science questions. We also need increased funding for laboratory studies that have specific planetary science goals.
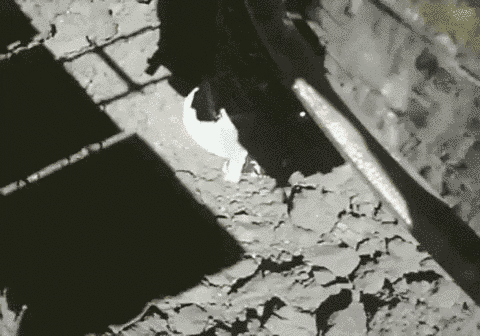
Are there important differences among the different types of small bodies?
Asteroids receive spectral classifications according to the amount of light they reflect. These classifications usually tell us something about their surfaces, but how important are those differences? Are there common origination processes shared by main-belt asteroids, Trojan asteroids that share Jupiter’s orbit, comets, moons of outer planets, and trans- Neptunian objects?
Questions like these will hopefully be answered by the New Horizons extended mission and especially by Lucy, a NASA mission that will visit 7 Trojan asteroids from 2027 to 2033. Did the Trojans form near Jupiter, or did they form in the Kuiper Belt and get flung inward during the migration of the giant planets long ago? Do their surface characteristics indicate a common formation mechanism?
What are the processes that dictate the orbital dynamics of interstellar objects?
Oumuamua, which was discovered passing through our solar system in 2017, has an elongated shape, and it is accelerating along its trajectory. However, it does not have a discernible coma nor is it releasing gas and dust tails, which makes this interstellar interloper very mysterious. The path of comet Borisov, discovered in 2019, mimics that of an interstellar object, and it has properties similar to comets in our own solar system. Observations of such objects are possible with ongoing observations by the Pan-STARRS ground-based telescope and are expected to increase after 2022 when the Vera C. Rubin Observatory (originally the Large Synoptic Survey Telescope) becomes operational. Unique interstellar objects could be studied up close in the future with the European Space Agency’s rapid-response Comet Interceptor mission.
—MAITRAYEE BOSE is a cosmochemist and assistant professor at the Arizona State University School of Earth and Space Exploration. She is on the steering committee of NASA’s Small Bodies Assessment group.

The outer planets of Jupiter, Saturn, Uranus, and Neptune as well as their satellites and rings offer a breadth of scientific opportunities for every discipline of planetary science. Whether you’re an atmospheric scientist studying storm dynamics, a geologist examining diverse terrains that include active volcanoes and geysers, or an astrobiologist studying the origin and evolution of life, there’s something for everyone.
It’s no surprise, then, that the fundamental science goals for the outer planets are representative of those of planetary science as a whole. In August 2019, NASA’s Outer Planets Assessment Group (OPAG) determined in preparation for the next decadal survey that exploring the outer planets can help answer the following overarching questions:
What is the distribution and history of life in our solar system?
How do planetary systems form and evolve?
What present-day processes shape planetary systems, and how do we get such diverse worlds?
A version of these questions can be found in the past two decadal surveys, represented as “cross-cutting themes” for all of planetary science. In fact, they also show up in precursor reports dating all the way back to 1965. These long-standing goals show the remarkable consensus that planetary scientists have when it comes to high-level goals for solar system exploration.
Outer-planets research is also relevant to endeavors beyond planetary science. The structure of the outer solar system serves as a prototype for exoplanetary systems, and the processes that shape those worlds help us explain similar processes on Earth. As a testament to the diversity of the outer planets, many research topics pursued by scientists studying the outer planets have synergy with other NASA science divisions: astrophysics, Earth science, and heliophysics.
THE PAST 10 YEARS
Outer-planets science during the past decade was led by NASA’s Cassini mission at Saturn, which ended in 2017, and Juno, which has been exploring Jupiter since 2016. During Cassini’s extended mission, the spacecraft flew in a polar orbit that allowed it to take detailed measurements of Saturn’s gravity field. Juno is doing the same at Jupiter, and data from both missions are being analyzed to determine the presence and size of a potential rocky core underneath the gaseous layers we see from space. Learning more about the cores will tell us how those planets formed and grew, capturing hydrogen and helium gas in our solar system’s protoplanetary nebula.
Additionally, Cassini and Juno have pointed radio antennas at their planets to measure microwave emissions from Saturn and Jupiter. Cassini used its telecom antenna to measure ammonia in Saturn’s upper troposphere, while Juno has dedicated antennas to sense ammonia and water deep in Jupiter’s atmosphere. So far, analyzing the Juno data has revealed the distribution of ammonia in latitude and depth below the colorful cloud tops. These measurements help us understand how the meteorological processes on Saturn and Jupiter work. One eventual goal for both planets is to measure the bulk abundances of volatile molecules such as water and ammonia, which will give us clues on how far from the Sun and how quickly these planets originally formed. We know from studies in the past decade that planets can significantly migrate toward and away from the Sun, which means that the 4 giant planets may not have formed where they are today.

THE NEXT 10 YEARS
During the next decade, NASA will send 2 missions to the outer solar system: Europa Clipper and Dragonfly. Europa Clipper will make repeated flybys of Jupiter’s moon Europa, while Dragonfly will land on and then fly around the surface of Titan. The European Space Agency’s JUICE ( JUpiter ICy moons Explorer) mission will investigate Ganymede, Callisto, and Europa. All 3 of these missions have a focus on determining whether life could have arisen beyond Earth.
Other missions to the outer planets have also been proposed. The main job of the decadal survey will be to prioritize specific science goals and evaluate which missions will best address these goals. The future mission concepts fall into 4 broad themes.
Surveys of Uranus and Neptune: Each of the ice giants has only been visited once—by Voyager 2. A spacecraft in a polar orbit of either world would allow us to examine the planet’s interior, and diverse moons and complex ring systems promise new discoveries like those made by the Cassini mission at Saturn. The current decadal survey recommended starting work on a Uranus mission after a Europa mission was greenlit.
Astrobiology: Following the Europa Clipper mission, a Europa lander would examine the surface for signs of life. A dedicated Enceladus mission could directly sample plume material spewing into space.
Atmospheric probes: Probes at Saturn, Uranus, and Neptune are needed to complete the noble-gas and volatile-species survey started by the Galileo Probe at Jupiter in 1995. A second Jupiter probe would also be helpful since the Galileo Probe entered an anomalously dry location, its measurements of volatiles were inconclusive, and Juno’s measurements can benefit from an in-situ validation.
Long-duration surveys of active worlds: We need long-term data on the active phenomena that shape worlds in the outer solar system, including up-close looks at the surfaces of Enceladus, Triton, and Io, the dynamic atmospheres of the giant planets and Titan, and the rings of each of the 4 giant planets. In addition to missions that visit these destinations, a space telescope dedicated to solar system targets would ensure that active worlds like these are observed regularly without large gaps.
The decadal survey is a truly major undertaking and a good occasion to remind ourselves that even with a diversity of destinations with many mission possibilities, we are all unified under common long-standing goals, and the heart of the survey’s task is to recommend the best strategy to answer those fundamental goals. Some of the outer-planet missions will last decades and span multiple generations of scientists, so such strategic planning is also important in shaping the careers of the next generation of scientists. No matter what the recommendations become, the outer planets offer something for everyone, and the decadal survey is sure to illuminate a path toward our next big discoveries.
—KUNIO M. SAYANAGI is an associate professor at the Hampton University Department of Atmospheric and Planetary Sciences.
Let’s Go Beyond The Horizon
Every success in space exploration is the result of the community of space enthusiasts, like you, who believe it is important. You can help usher in the next great era of space exploration with your gift today.
Donate TodayThe Planetary Report • March Equinox
Help advance space science and exploration! Become a member of The Planetary Society and you'll receive the full PDF and print versions of The Planetary Report.